Research
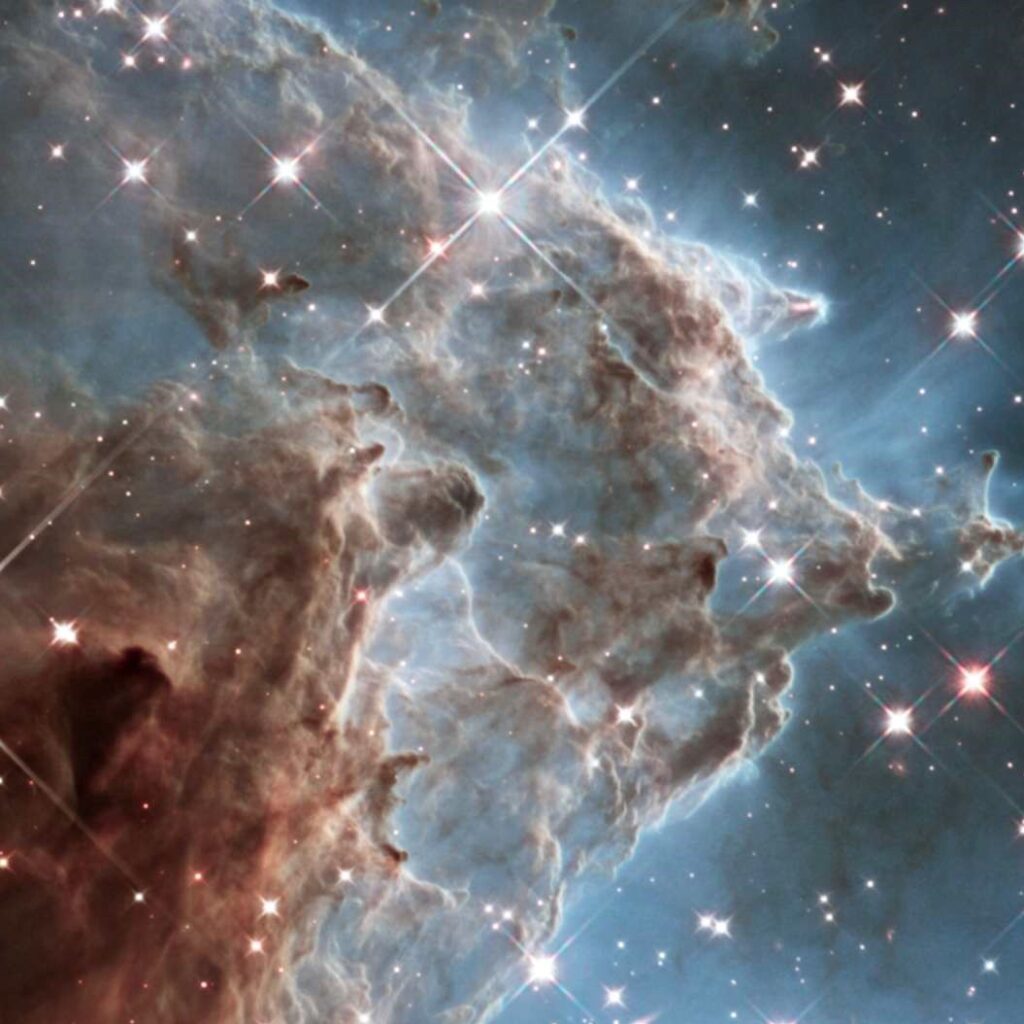
Neutrino Astrophysics
Neutrino astrophysics is a branch of physics that studies the most-energetic phenomena in the Universe through the observation of neutrinos of cosmic origin. In this regard, neutrinos can provide unique insights on the production and acceleration processes in the sources, even if they are very distant or obscure objects.
Astronomy has been traditionally done with electromagnetic observations. However, at very-high-energies (> TeV) photons (gamma-rays) get absorbed after the interaction with the extragalactic background light, therefore reducing the observation range. For instance, at hundreds of TeV only sources in the Milky Way can be detected. Neutrinos, on the contrary, can travel unabsorbed from long distances.
High-energy astronomy with cosmic rays (ionized nuclei) is also possible. The main drawback in this case is that the trajectory of cosmic rays from the source to Earth is bent by the magnetic fields beyond Earth and therefore the directional information is lost. Only at ultra-high-energies (above 1 EeV or 1019 eV) cosmic rays deflection is small enough to point back to the source. On the contrary, neutrinos have the advantage that they are neutral, so cosmic neutrinos can be reconstructed with sub-degree resolutions.
The main disadvantage of the neutrinos is that they have a small cross-section so to get a detectable flux at Earth it is required large detection volumes, of the order of km3, to be able to gather enough statistics to reach a detection threshold of 5σ.
Other cosmic messengers that play a key role in high-energy astronomy are gravitational waves (GWs). First detected in 2015, GWs appear as a consequence of massive objects moving with high energies. GW detections can be combined with neutrino and other electromagnetic and cosmic ray observations offering a new way of exploring the Universe that is coined as multi-messenger astronomy[1]. In this fashion, many experiments around the globe with a large sky coverage and mostly continuous data taking, provide the tools necessary to study the potential correlations of these messengers (see Figure 1).
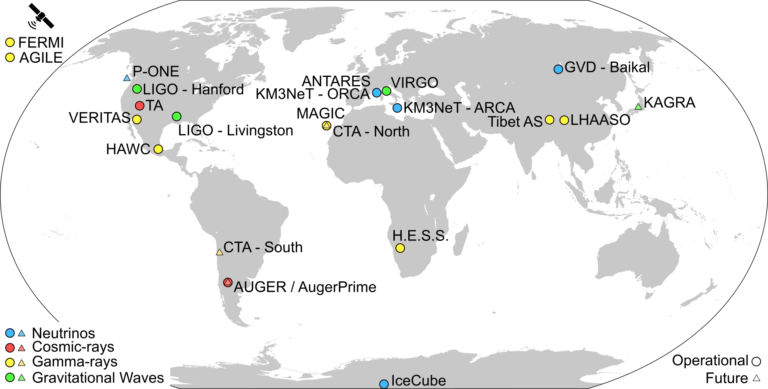
Figure 1: Distribution of the main observatories of different messengers around the globe.
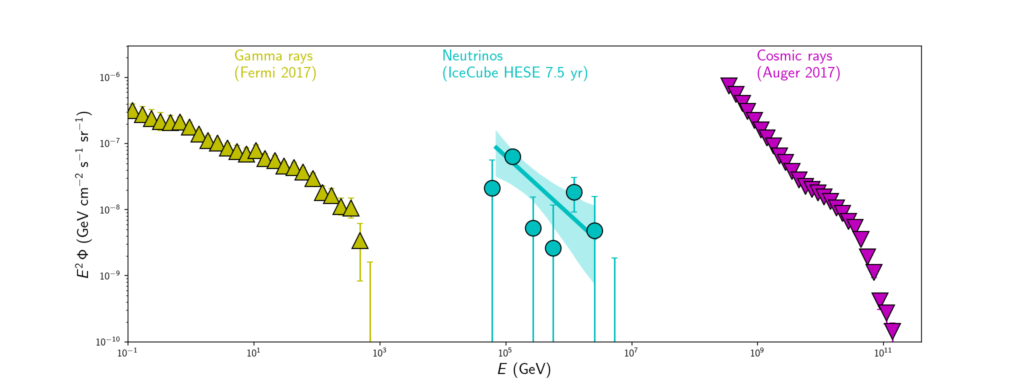
Figure 2: Observed fluxes at the highest energies of gamma ray, neutrino and cosmic rays.
Probing the connection among those messengers is not trivial, but combining their inputs significantly increases the discovery potential. This is what transient analyses can achieve, by adding the time constraint to their potential correlation. During the following decade many experiments will coordinate their observations in real time, likely achieving the discovery of the first high energy neutrino source.
Today we are in the Multi-messenger era.
[1] “Multimessenger Astronomy with Neutrinos”, Francisco Salesa Greus and Agustín Sánchez Losa, Universe 2021, 7(11), 397. DOI:10.3390/universe7110397 [arXiv:2110.11817]
[2] “Science with Neutrino Telescopes in Spain”, J.J. Hernández-Rey et. al., Universe 2022, 8(2), 89. DOI:10.3390/universe8020089